Demystifying Electromagnetics, Part 3 – Magnets
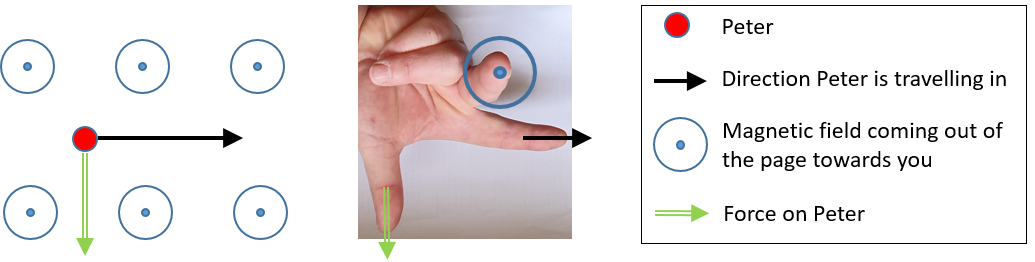
In Part 1 and Part 2 we explored the electric field, a force field created by charges that only acts on charges. Magnetic fields are similar in some ways, in fact as this blog series goes on we’ll see that the electric field and magnetic field are 2 sides of the same coin (it’s called ‘Electromagnetics’ for good reason). How best to demystify magnets and the magnetic field? Let’s pose some basic questions…
What Generates a Magnetic Field?
Answer: A moving charge
It really is as simple as that. An isolated charge (let’s assume it’s a single proton) will always generate an electric field. As soon as it starts to move however it will also generate a magnetic field. Whereas the electric field ‘flows’ outwards from the proton, the magnetic field ‘flows’ around the moving proton:
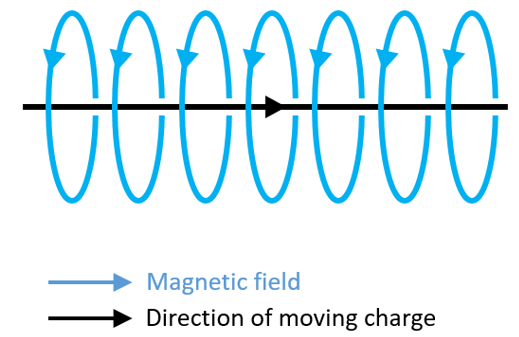
The further away from the moving proton, the weaker the magnetic field, but the field always circulates, it always loops around without a beginning or an end.
What Does a Magnetic Field Do?
Answer: It exerts a force on a moving charge
Similar to the electric field that exerts a force on a charge, a magnetic field will also exert a force on a charge but only when that charge is moving. One might have assumed that the direction of that force is in the direction of the blue arrows indicating the direction of the magnetic field. After all, that’s how the electric field force worked, right? Not quite, the direction of the force is at 90 degrees to both the magnetic field direction and the direction that the proton is moving in.
Consider a single lonely proton, let’s call him Peter, just sauntering along minding his own business, when suddenly he walks into a magnetic field:
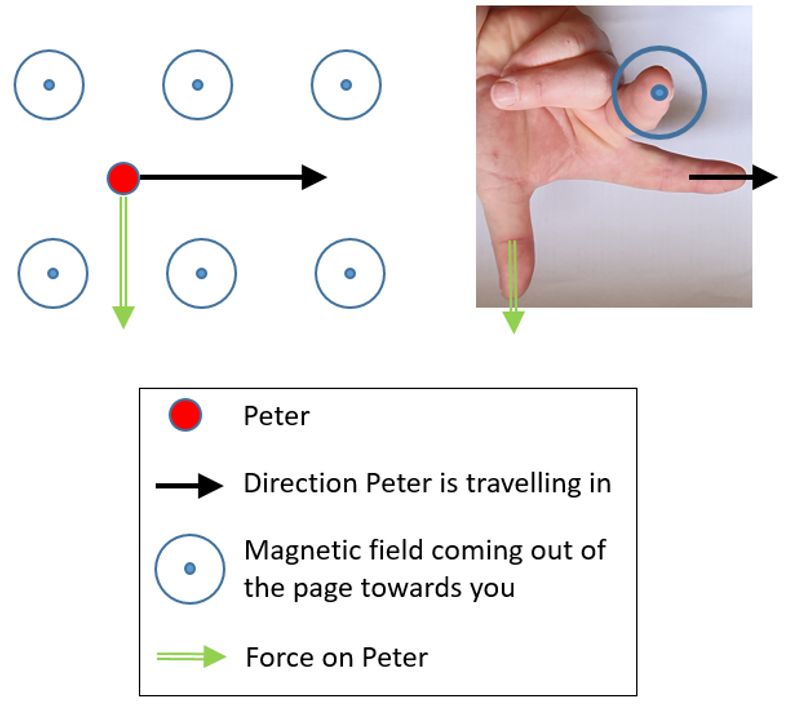
Peter would be forced downwards. Due to his now changed direction he’d then be forced sideways, then up, the right etc. In fact he’d end up going in a circle.
The direction the force is applied, given the direction the charge is moving in and the direction of the magnetic field, can be found using the right hand rule. One’s thumb is the result of the dot product (the force), the index and middle finger point in the directions of the velocity and magnetic field vectors. It’s worth noting that the right hand rule will give the direction of the force a +ve charge would experience. If instead it was a lonely Erik the electron, a –ve charge, the direction of the force would be reversed.
One comment about Peter, sure he creates his own magnetic field because he is moving, but in the above example the external magnetic field that ‘comes from somewhere else’ is so big that we can ignore Peter’s feeble field.
Whereas it might seem strange that the force exerted is at 90 degrees to the direction of travel, a helpful analogy might be the lift force a wing experiences as it travels through a fluid.
How Large is the Force?
Answer: it depends on the amount of the charge and the speed it is travelling
The force a charge experiences due to an electric field = Eq, where E is the electric field strength (N/C) and q is the charge (C). The force a charge experiences due to a magnetic field = q(v X B) where v is the velocity vector of the charge (m/s), B is the magnetic flux density vector (Tesla) and X is the cross product of those 2 vectors. If the velocity and magnetic flux are at 90 degrees to each other, the force exerted is at a maximum.
The Lorentz Force
Combining these, the total force a charge experiences due to both electric and magnetic fields is called the Lorentz force:
F = q(E+(v X B))
If v is zero, there’s only the electric field contribution. Generally, the bigger the charge q the bigger the force that will be exerted on it.
Going back to our analogy table, it’s interesting to note that whereas it’s the electric field strength E that contributes to the Lorentz force, it’s the magnetic flux density B that provides the magnetic contribution to that overall force not the magnetic field strength H. Well I found that interesting.
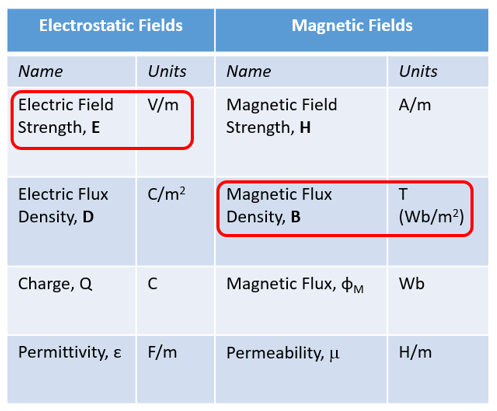
Meet Petronella
The lonely Peter is joined on his walk by his proton friend Petronella. Their magnetic fields interact but are Peter and Petronella attracted or do they repel each other? It depends on which direction each of them is walking in. What if they are walking in the same direction?
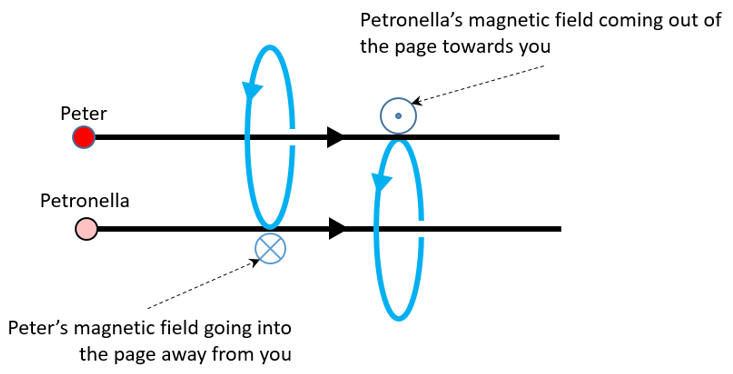
Peter’s magnetic field sweeps over Petronella, and vice versa. The circled cross notation indicates that the field is going away from you (like seeing the fletched end of an arrow as it flies off away from you).
Using the right hand rule we can determine the direction of the force Peter exerts on Petronella, and vice versa:
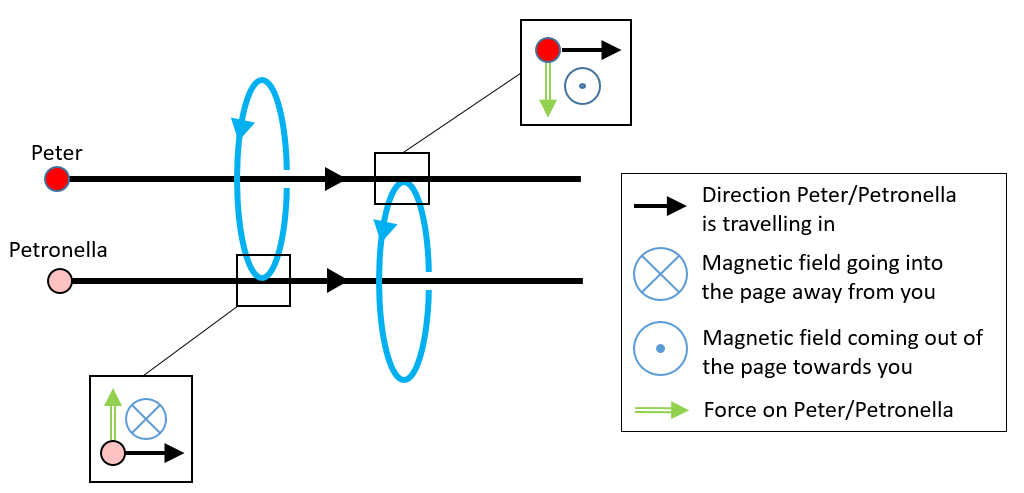
They attract, the force arrows point towards each other. As you’d expect, they’re both walking in the same direction, they must like each other 🙂
If they are travelling in opposite directions, the force arrows point away from each other, they would repel.
However…
Peter and Petronella not only generate a magnetic field due to them moving, they also generate an electric field due to them having a net +ve charge, and like charges repel:
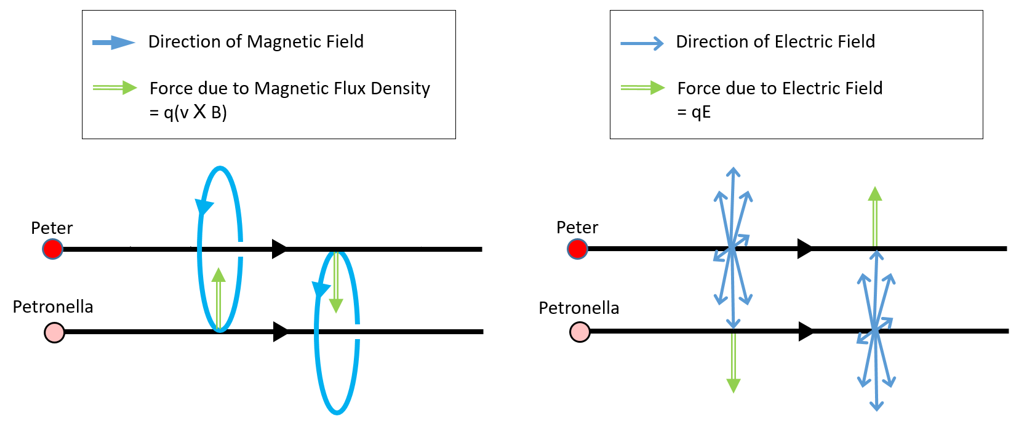
The repelling force due to their electric fields is just a function of their charge. The attractive force due to their magnetic fields is due to both their charge and their velocity. Only when the magnitude of that velocity is the speed of light do those 2 forces balance and the proton (beams) remain parallel.
Conducting Wires are Charge Neutral
Consider a copper wire just sitting there, no current flowing through it. It is charge neutral in that the number of electrons balance the number of protons. It does not have a net charge. It does however have ‘free’ electrons. Connect that wire to a battery and those free electrons start to flow as we covered in Part 2.
Even when those electrons are flowing the wire still has a neutral charge, there is still not an excess (or deficit) of electrons. For every electron that leaves the wire at its battery terminal, another one joins from the other terminal.
So, due to the movement of charge there is a magnetic field generated, but because there is no net charge, there is no electric field.
Going from Beams to Conducting Wires
Instead of Peter wandering through space as a beam, let’s constrain him in a conducting wire. Let’s also loop that wire so Peter just goes round and round and round as part of an electric current, I. Also best to consider Peter as one of the billions of electron holes rather than a moving proton as it’s those electron holes that ‘move’ in the direction of the current, the protons remain bound to the atomic nucleus.
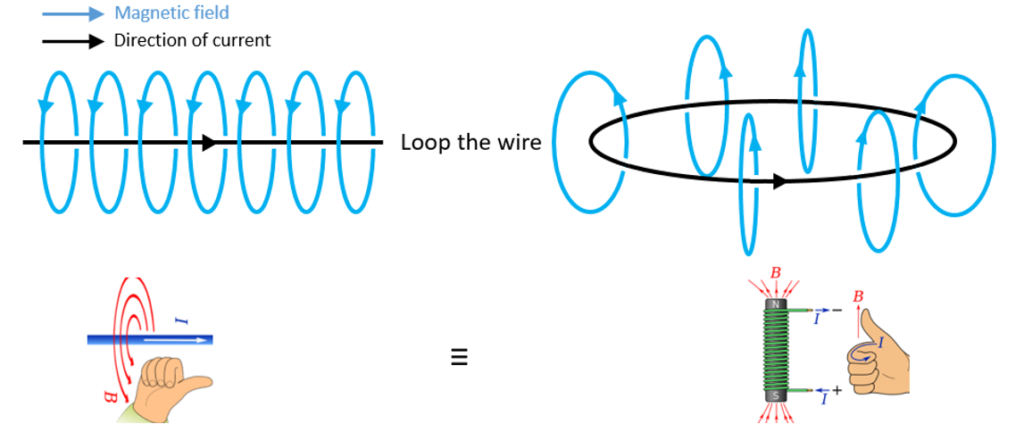
As we saw with Peter and Petronella, the 2 wires that both have current flowing through them in the same direction will attract each other due to the magnetic component of the Lorentz force, even when they are looped:
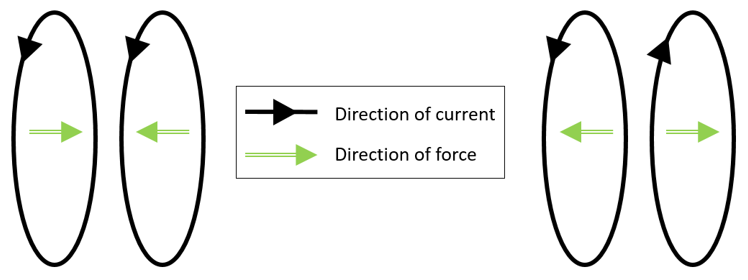
A spiral of wire is akin to loots of loops of wire stacked on top of each other, massively increasing the strength of the resulting combined magnetic field. Superposition in action again.
The attraction is just the force being applied to all the charges and those charges, constrained as they are within the solid conductor, ‘drag’ that conductor along with them.
The magnetic field generated by a single loop, or spiral, looks suspiciously similar to that generated by a permanent magnet. But magnets don’t have current in them, right?
What is a Permanent Magnet?
Answer: It’s kind of like a conducting loop
Not a very good answer, sorry. Let’s come back to this question a bit later, see if the answer (as vague as it was) will make any more sense.
Do Atoms Generate a Net Magnetic Field?
Answer: Some of them do
An atom consists of a nucleus with electrons spinning around it (is one way of conceptualising them). As we’ve seen with the conducting wire loop that generates a magnetic field, same is true for atomic electrons:
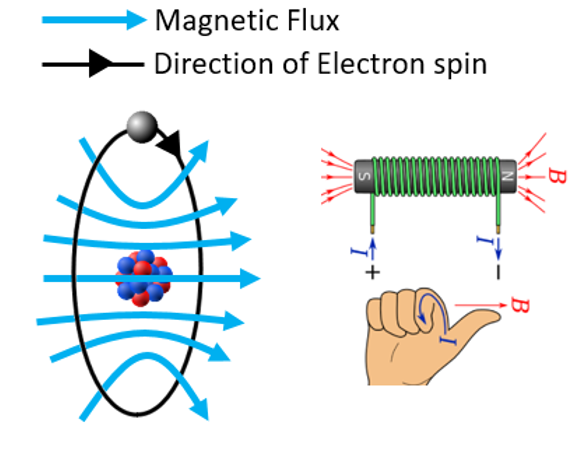
Note that the right hand rule is formulated for the flow of +ve charge, that’s the notation for current flow direction. As we’re now talking about a spinning electron, a –ve charge, the right hand rule is reversed, thus the contradiction between the direction of the electron spin (left) and the direction of the current flow in the curled fingers (right).
For most elements, these electrons come in pairs, each spinning in a different direction so as to cancel out the net magnetic field:
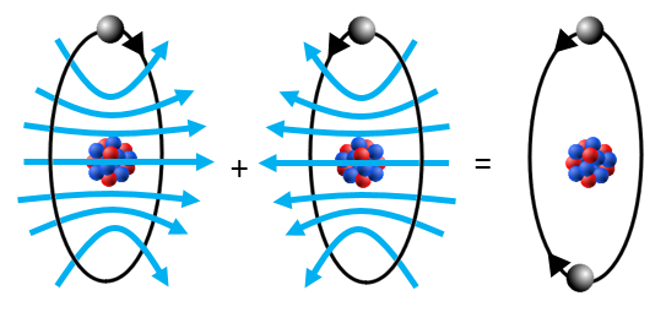
Some elements have an unpaired outer electron, the common ones being Iron, Nickel and Cobalt, the so-called ferromagnetic materials. Each individual atom therefore creates its own mini magnetic field. When the atoms are oriented randomly, these fields will again cancel each other out:
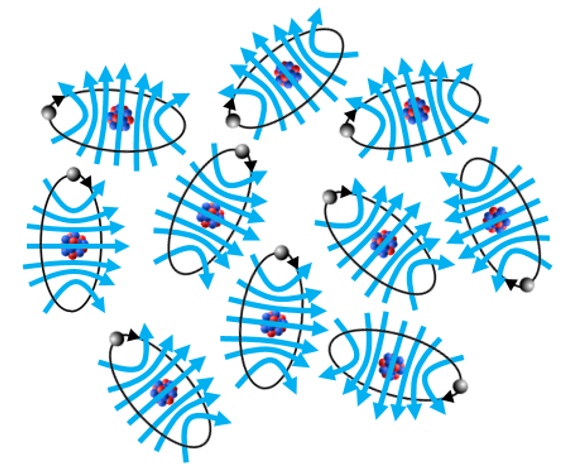
If all the atoms are all aligned, their individual magnetic fields combine, superimposing to create a strong net magnetic field. The material then becomes magnetic:
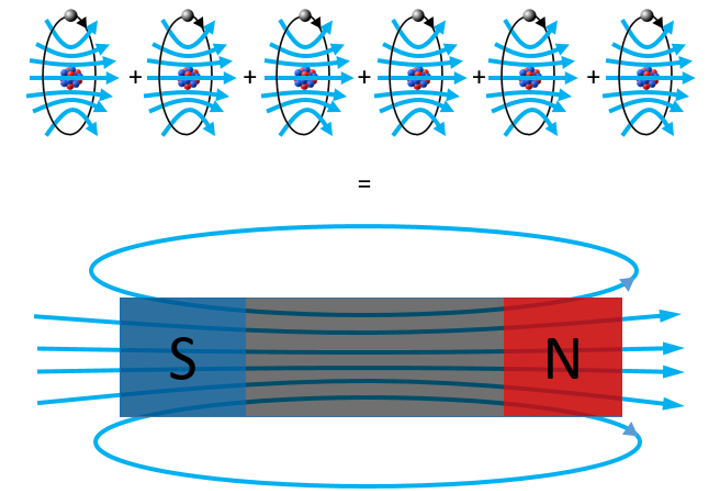
How are Permanent Magnets Made?
Answer: Hit them with a hammer
I’m not being facetious, this is partly true. When a magnetic material is placed in a magnetic field, it can itself become magnetised. This external magnetic field aligns all the atoms causing the material itself to become magnetised, either permanently or until the material is removed from that magnetic field. Hitting it with a hammer whilst in a magnetic field, even stroking the material over the surface of another magnet, will align the atoms and create the magnet.
What is a Permanent Magnet?
Answer: It’s kind of like a conducting loop
Let’s try this one again. Imagine we could see all the aligned atoms in a bar magnet, looking at the south pole so that the magnetic field was going away from us into the page. I’ve simplified things just to show the unpaired outer electron spin:
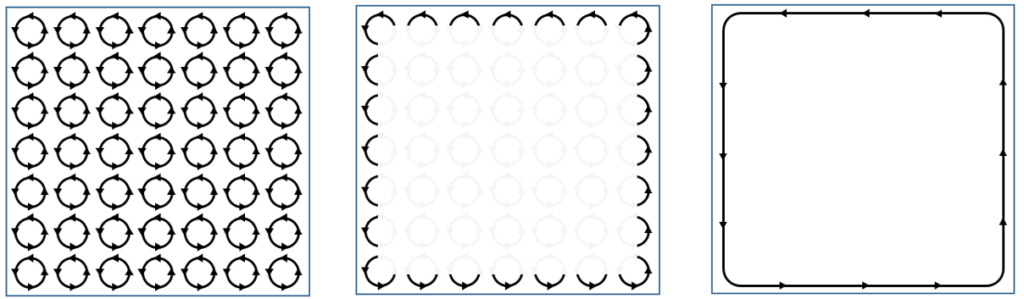
The electrons within the material away from the periphery, due to their spin direction being opposing where they abut, cancel each other out in terms of the net charge flow. What’s left is an effective current flow around the periphery of the material that is analogous to a conducting wire loop. That is why the resulting magnetic field from a permanent magnet is arguably identical to one from an equivalent conducting loop of wire.
Why do Magnets Attract or Repel?
Considering a magnet is in effect a conducting wire loop and we’ve seen how, due to the Lorentz force, wire loops can either attract or repel each other, the question is already answered! The charges contained in each magnet, or magnetic material, are forced together or apart. As the charges cannot escape the material, the entire solid is dragged along instead.
Anecjoke
An anecdote this time. Ships tend to have a lot of ferrous material in them, be it the hull itself or the engine, rivets etc. These parts may themselves be magnetised either during their construction or, when the ship sails in a single direction for a length of time, by the earth’s magnetic field.
Despite advances in satellite GPS navigation, it is still a legal requirement that a ship has a magnetic compass installed. The compass will align itself with any magnetic field it finds itself in. Whereas the intention of the compass is to align with earth’s magnetic field, it will be affected by the ship’s own field.
To compensate for the local effect of the ship’s magnetic field, the binnacle that houses the compass is fitted with soft iron components whose size and position can be calibrated so as to cancel out the ship’s field resulting in the compass giving a true indication of magnetic north. Such components may include the wonderfully named ‘Kelvin’s Balls’ and ‘Flinders Bar’.
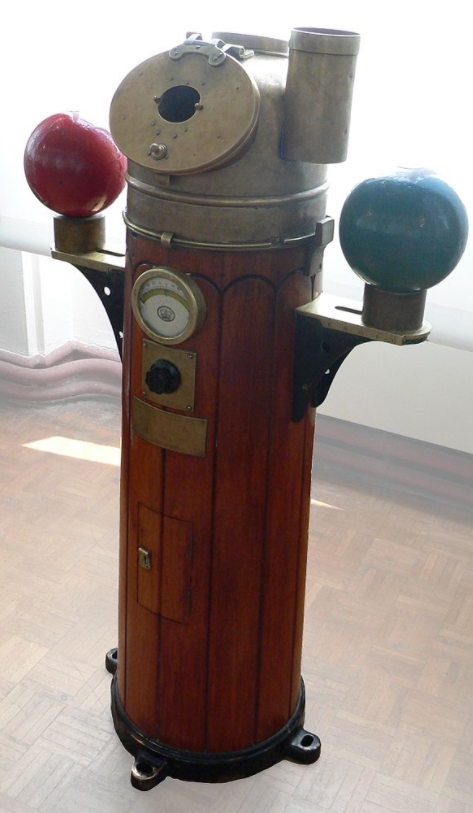