New accuracy study opens the door to CFD led clinical trials
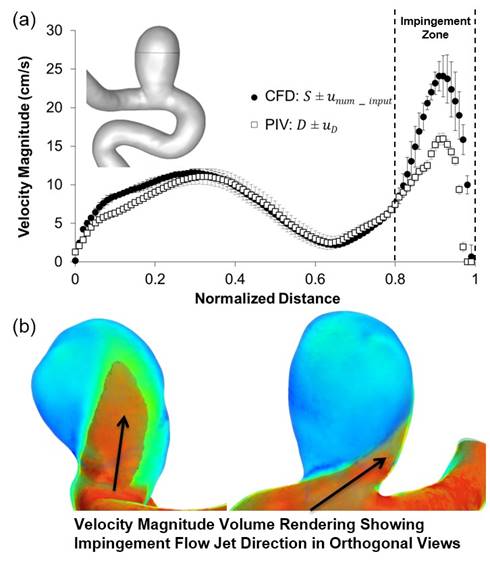
Computational fluid dynamics (CFD) is a vital tool for the research of blood flow dynamics in the human cardiovascular system as well as other biological flow phenomena. Recently, CFD has garnered increasing interest as both a clinical and medical device innovation tool. However, before CFD can be used as a credible alternative to in vivo or in vitro flow analysis, validation is required to assess if a candidate CFD solver can accurately capture the physics of the biological flow in question. There have been ongoing efforts from the U.S. Federal Drug Administration (FDA), in collaboration with the American Society of Mechanical Engineers (ASME), to develop verification and validation guidelines for computational modeling of medical devices, known as the ASME Verification & Validation 40 Standard (ASME V&V 40 – currently under development). These guidelines, however, do not provide steps on how to perform validation. Thus, there are currently no validation protocols to quantify the credibility of a CFD solver based on its accuracy in its intended context of use.
For this purpose, a team of researchers at the University at Buffalo consisting of Nikhil Paliwal, Robert Damiano, Nicole Varble, Vincent Tutino and Zhongwang Dou under the guidance of Professor Hui Meng from the Department of Mechanical and Aerospace Engineering, and Dr. Adnan Siddiqui, from the Department of Neurosurgery, developed a validation methodology to quantify the accuracy of CFD solvers in the biomedical context of use. Their proposed validation methodology is based on the concepts of the ASME V&V 20 Standard. Instead of showing the difference between the simulation and experimental data (a commonly used validation approach), their validation methodology identifies all sources of error in CFD simulation and validation experiments. Based on these sources, the CFD accuracy is then quantified in terms of the “model error,” which represents the error in the solver due to its modeling assumptions. The model error is expressed as the combination of validation error and uncertainty, which includes the error due to numerical discretization, input parameters to the CFD solver and errors in the experimental measurements (figure 1).
Figure 1: Flow chart showing sources of error in CFD model and PIV experiments used to quantify the CFD model error
To demonstrate the application of their proposed validation protocol, Paliwal et al. simulated physiologically pulsatile blood flow in a patient-specific intracranial aneurysm (IA) model using Siemens PLM Software’s STAR-CCM+® version 10.02. To obtain experimental validation data, they performed 2D particle image velocimetry (PIV) and measured the velocity flow field on two orthogonal planes in the IA sac. Furthermore, they performed optimization of STAR-CCM+ solver parameters like grid size, temporal resolution and solver order of accuracy to identify the optimum STAR-CCM+ settings for solving flow in the IA model.
Figure 2: Velocity magnitude in STAR-CCM+ and PIV plotted along the validation line; error bars represent uncertainties in CFD and PIV, respectively
Error and uncertainties were calculated using the validation protocol. Also shown is the flow jet impinging on the distal wall of the IA, which was the reason for the large differences between STAR-CCM+ and PIV in the impingement zone (dotted vertical lines).
The validation results showed that the STAR-CCM+ solver had a model error of 5.63±5.49% in predicting flow along the line of intersection of orthogonal planes inside the IA sac (validation line). Figure 2(a) shows a pointwise plot of the velocity magnitude along the validation line in the STAR-CCM+ and PIV results. The error bars in CFD show the uncertainty in simulation due to mesh discretization error and error in the input parameters. The error bars in PIV represents uncertainty in the experimental measurements. Further analysis of the aneurysmal flow field revealed that a strong inflow jet was present in the IA sac which was hitting the distal wall of the aneurysm, as shown in the volume rendering of velocity magnitude in figure 2B. This impinging flow resulted in high velocities and velocity gradients in and around that region. Consequently, the STAR-CCM+ model error was higher in this region (impingement) where the flow is hitting the wall at higher velocities, as shown by the dotted vertical lines in figure 2.
In conclusion, this study provides a rigorous validation approach that quantifies the “true” accuracy of CFD solvers in the biomedical context of use. The model error represents the error in simulation results due to the solver’s modeling assumptions. The application of validation protocol on the test IA model showed a model error value of 5.63±5.49% in the STAR-CCM+ solver. This study provides a path for CFD solvers to perform a systematic validation and quantify their credibility in the intended biomedical context of use.
“We hope that this methodology can become a benchmark for validation of CFD solvers in the biomedical industry,” said Professor Hui Meng, researcher, University of Buffalo Department of Mechanical and Aerospace Engineering.
_____
Reference
Paliwal N, Damiano RJ, Varble NA, Tutino VM, Dou Z, Siddiqui AH, Meng H. Methodology for CFD Validation for Medical Use: Application to Intracranial Aneurysm. Journal of Biomechanical Engineering. 2017.
Note
This study has been published in the ASME Journal of Biomechanical Engineering. The detailed paper can be found here:
http://biomechanical.asmedigitalcollection.asme.org/article.aspx?articleid=2653365