Future electric cars must focus on battery, architecture development
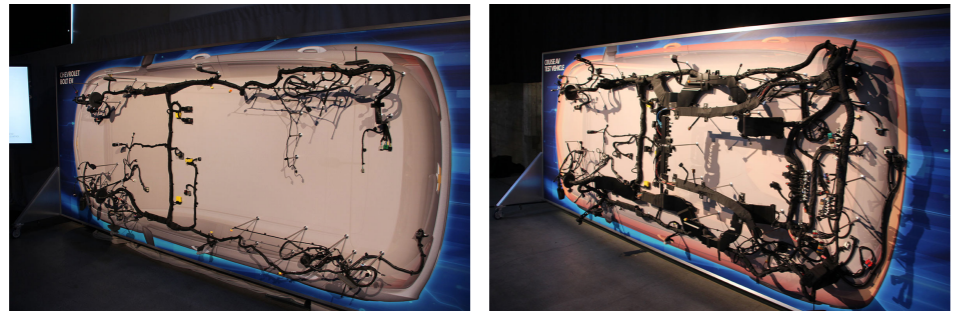
Electric cars are on display in Arizona where Waymo formally launched its self-driving taxi service — thus, the race to create the first genuinely commercially-viable level 4 autonomous vehicle has begun.
This keyword, “commercially-viable,” represents a massive change from the autonomous vehicle test fleet that the majority of automakers and other companies have been running for many years now. It describes, first and foremost, the importance of reliable driverless future electric cars in any traffic condition, plus the need for sufficient driving range and in-cabin experience for passengers.
It’s interesting to see how silently electrification and autonomous driving technologies are about to collide within a vehicle as companies design the cars of tomorrow. According to an analysis conducted by Securing America’s Future Energy (SAFE), 58 percent of autonomous, light-duty vehicle retrofits and models are built over an electric powertrain, while a further 21 percent use a hybrid powertrain.
Electrification is indispensable for autonomous vehicles because of such factors as changing environmental regulations regarding fuel efficiency and emissions, rapidly decreasing total cost of ownership and the projected increase in urbanization — 55 percent of those living in cities in 2018 vs. a projected 68 percent in 2050.
One of the biggest challenges of bringing the autonomous driving and electrification to the vehicle is the addition of high power consumption electronics that enable autonomous driving and, thus, drains the heart of future electric cars – the battery.
The battery-draining electric powertrain
Level 4 autonomous vehicles are likely to have more than 30 sensors, multiple engine control units (ECU) and sensor fusion boxes; all these electronics, in a pure electric vehicle, can easily reduce battery drive range up to 35 percent and significantly add to driving range concerns.
Similarly, for autonomous fleet operators, revenues are directly tied to the miles driven per charge. Such a steep range reduction, due to autonomous functionality, pose significant business challenges. Finding a battery pack that can deliver same energy with 35 percent less volume at a cost similar to today’s state-of-the-art battery is very challenging, and likely won’t be possible in the near future.
Compensating for range reduction with autonomous driving functionality is a big challenge, but unique design optimization opportunities exist if one can exploit autonomous vehicle usage profiles and its impact on the electric powertrain. In contrast to human driving, machine driving can be designed to operate more smoothly and efficiently, especially in urban settings. For example, as vehicles become more connected with other vehicles via smart cities, autonomous driving is expected to intellectually evolve. Vehicles may slow down or accelerate based on driving conditions unseen by human drivers, thus significantly increasing electric powertrain energy efficiency.
Simulation tools have shown the impact of such driving profiles on electric vehicle drive range and found that connected autonomous electric vehicles can achieve approximately an 80 percent driving range, even with high-power consuming autonomous driving electronics.
Also, changes in driving patterns reduces heat generation from the battery, motor and power electronics – key components of an electric powertrain. Since temperature is a major life-limiting stressor, these component suppliers and OEMs can engineer and integrate a more robust electric powertrain with less bulky thermal management strategies. Of course, if the overall volume for power electronics and motors is lowered due to such engineering exercises, OEMs can budget more space for cells in a pack and thus increase range.
The electrical electronics (EE) architecture
The EE architecture on future electric cars will be more complex for autonomous electric vehicles than non-autonomous electric vehicles. As part of an investor presentation in November 2017, GM described that to add autonomous functionality to the Bolt, it added 40 additional sensors and 40 percent new hardware content compared to the non-autonomous version. This addition creates significant packaging, weight and architectural challenges in the electrical and electronics domain. The two images below, a Chevy Bolt low voltage harness on the left and the Chevy Bolt harness for autonomous on the right (test vehicle not yet in production) captures the challenges with autonomous electric vehicles perfectly.
Autonomy and electrification demand significant changes to the vehicle’s architecture. The electrical distribution system (EDS), the third heaviest system in the vehicle, will need to be evaluated with renewed scrutiny and focus on reducing the physical size and mass relative to its current form. Other considerations include the introduction of high voltages, higher levels of safety considerations and weight reduction in attempts to achieve maximum range. Finally, the architecture must be reconsidered to factor in elements like electromagnetic interference shielding, which can be difficult to bend and add weight.
Developing the car of tomorrow: evolving engineers and engineering
“Tomorrow’s cars will be safe, green and connected,” Mary Gustanski, Delphi’s vice president of engineering, stated at a 2017 UN event. “We’re going to see more electrification, and the electric car will merge with automated driving and the connected car.”
For driverless electric vehicle development, a key metric every major company is tracking and reporting is disengagement rate, which represents how many times a human had to take over in real-world testing. Real world testing is, however, a minor part of overall testing, validation and verification of future electric cars.
The sheer permutations and combinations of testing scenarios are forcing automakers to rely on virtual testing, which can also be leveraged to do the heavy work of design engineering, verification and validation. Virtual testing, validation and verification of autonomous vehicles must also cover impact on range and passenger experience in addition to the reliability of autonomous driving. This can help suppliers and automakers collaborate on every aspect of the new mobility experience. For instance, autonomous vehicle driving behavior as tested in a virtual world can be connected to the digital representation of the vehicle e-powertrain to evaluate and optimize powertrain energy efficiency and drive range.
Similarly, new mobility concepts can be easily evaluated and tested within a CAE framework to assess its impact on passenger comfort and safety. For example, machine driving will need to target vehicle dynamics that won’t jerk around passengers so they won’t get hurt or nauseated. Within this framework, virtual validation and simulation can significantly reduce the time to roll out new vehicles/models.
The auto industry and the tech industry must continue working cohesively. New engineers and engineering ways will be needed to support the rapid innovation and shorter development cycle without sacrificing safety and security. The digital twin framework offers a path.
As hundreds of automakers and manufacturers continue creating future electric cars, it will be crucial to think of both electric and autonomous together from the very beginning. Engineers and designers must account for challenges and opportunities that may pop up along the way; you can’t just slap sensors and add electronics as a plug-and-play option. If you wait until the end to bring everything together, changes can be costly and reduce a manufacturer’s ability to deliver a driverless electric vehicle to market.
Click here to learn more about electric vehicles.
About the author
Puneet Sinha is the automotive manager in the Mechanical Analysis division of Mentor, a Siemens business. In this role, Puneet is responsible for his division’s business strategy and market development for vehicle autonomy and electrification thrusts. Puneet has more than a decade of experience working on various aspects of vehicle electrification. Prior to joining Mentor, he worked at General Motors, where he led global research and development teams to solve issues with fuel cells and battery electric vehicles. He also worked at Saft, a Li-ion battery manufacturer. Puneet has a Ph.D. in mechanical engineering from Pennsylvania State University, has authored more than 20 highly-cited journal articles and has been awarded seven patents on fuel cell and battery system design and operational strategies.
Comments